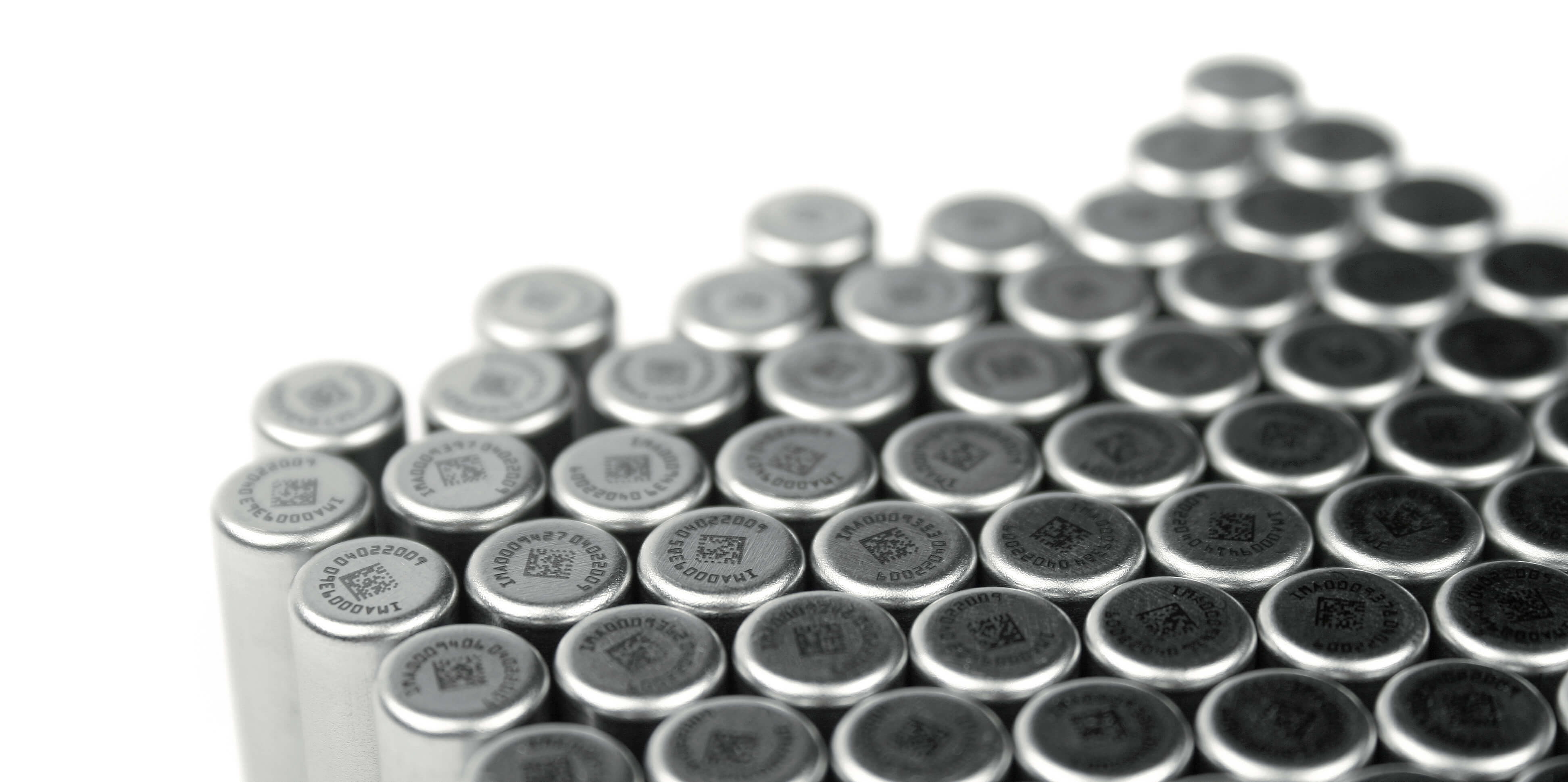
When faced with a patient exhibiting signs of infectious disease, time is of the essence. The faster a diagnosis can be made, the faster public health measures can be implemented and the appropriate treatment delivered. Molecular assays based on RT-PCR, RT-LAMP, and other amplification techniques have helped to markedly reduce diagnostic turnaround time and are increasingly viewed as the gold standard. However, many of the reagents used in these assays require freezing temperatures during storage and transportation. This poses a significant challenge as freezers are cumbersome, demand large amounts of energy, and are susceptible to failure when power sources are compromised.
With the cost of maintaining cold storage so high, point-of-care molecular diagnostics are often not available in remote communities, severely hindering public health efforts.
Developing reagents for molecular assays that are stable at room temperature is a big step towards improving access to gold-standard diagnostics. To this end, Twist Bioscience has teamed up with Imagene, a leading company in the field of room temperature biopreservation, to produce encapsulated synthetic RNA controls that are exceptionally stable at room temperature. These synthetic controls serve as a necessary positive control reagent in diagnostic assays. As such, this RNA can be used to verify and validate infectious disease assays in labs without the need for ultra-cold storage.
Here, we give a brief overview of how the extended stability of Twist’s RNA was achieved and what impact it may have on molecular diagnostics.
How stable is RNA at room temperature?
Not surprisingly, RNA is extremely unstable at room temperature. There are many reasons for this, including RNA’s basic structure. Unlike DNA, the ribose sugar in RNA has a 2’-hydroxyl group that can attack the phosphorus atom linking one ribonucleotide to another and break the bond holding them together1. In the presence of water, this attack is strongly favored, meaning RNA stored in aqueous solutions is very prone to breakdown.
One way to slow the rate of RNA degradation is to decrease molecular movements by keeping your sample in ultra-cold conditions (-20 or -80oC). Not only does this reduce RNA’s self-destruction, but it hinders enzymatic degradation of the RNA. However, even under freezing conditions, degradation does happen—albeit slowly—which may limit the shelf-life of RNA samples2,3.
Dehydration is another approach to improving RNA sample stability. Without water to catalyze the 2’-hydroxyl attack and to enable molecular mobility, RNA degradation is significantly reduced under anhydrous conditions. To maintain a water-free environment, though, researchers have to consider the atmosphere in which the dehydrated RNA is stored. Water is a significant component in typical atmospheric conditions and can rehydrate samples, ultimately leading to the demise of the RNA contained therein3.
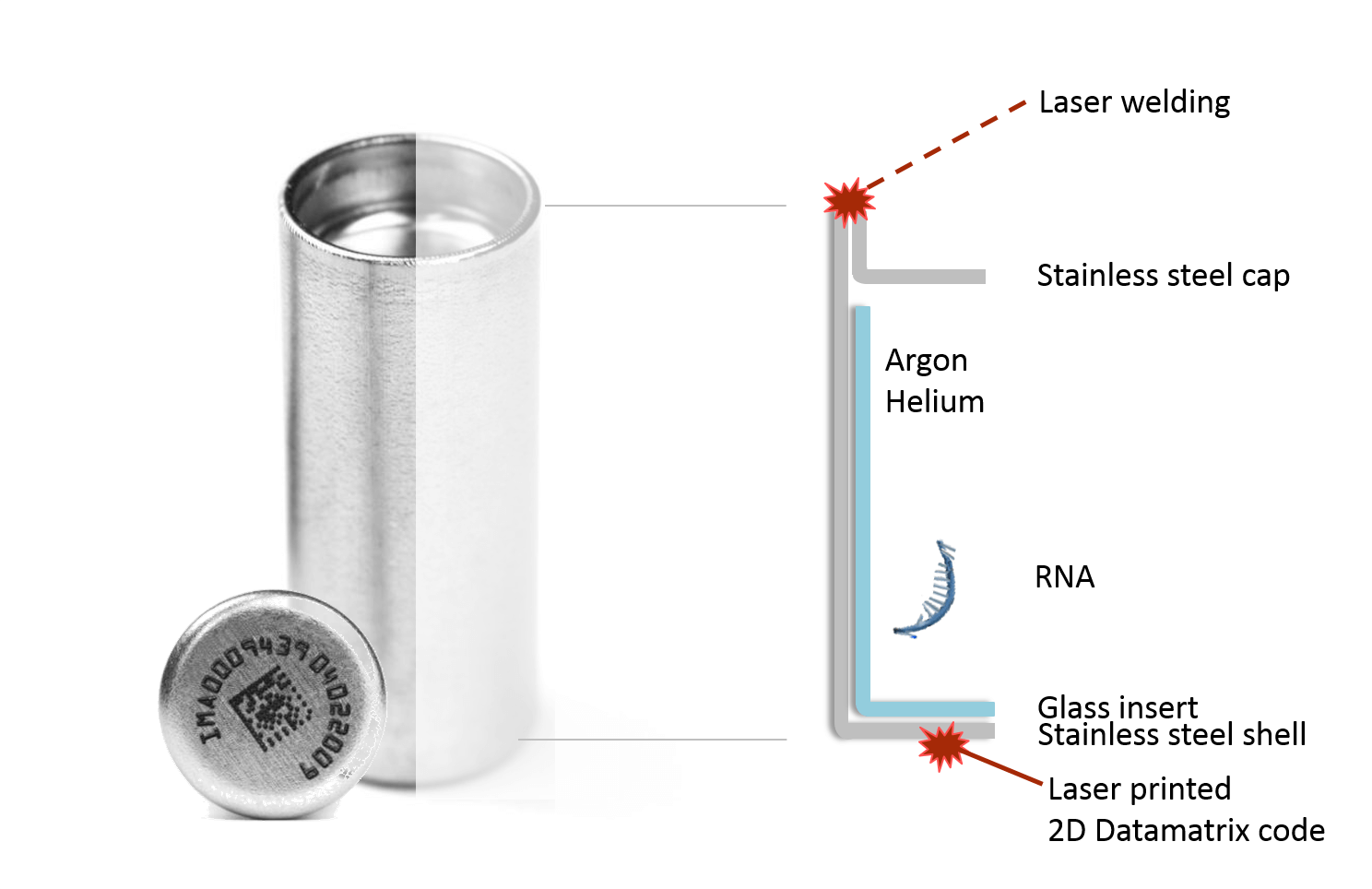
To extend the lifespan of RNA samples, Imagene developed a storage solution in which dehydrated RNA samples are stored in glass tubes and sealed inside stainless steel “minicapsules”. The atmosphere in these storage vessels is largely argon and helium with less than 1 ppm of oxygen and water4. Head-to-head comparisons suggest RNA samples stored in these minicapsules are at least as viable as samples stored in ultra-cold conditions5. Simulations also indicate that the encapsulated RNA is likely to be stable under room temperature for up to a decade2.
Stabilizing RNA for molecular assay development
Maintaining ultra-cold freezers is an expensive task. Not only is the upfront cost high, but these devices require exorbitant amounts of energy, occupy valuable laboratory space, and require constant monitoring in case power failures should occur. As a result, the number of freezers in a laboratory can be very limited. This finite amount of space available for ultra-cold storage often means labs have to order reagents on a rolling basis, taking care to never have more than can fit in the freezer. The cold-storage bottleneck limits a lab’s capacity for molecular testing, including critical testing used to identify pathogens like SARS-CoV-2. Reducing the need for cold-storage could have a transformative impact on molecular biology.
Twist Bioscience has been synthesizing RNA controls to help researchers avoid the dangers and variability associated with virus-derived RNA. Twist Synthetic RNA Controls are used to determine the limit of detection, monitor day-to-day test variations, and are listed on the U.S. Food and Drug Administration (FDA) website as positive control materials for SARS-CoV-26.
Whether it is in research or point-of-care applications, positive controls are critical. But for all the reasons listed above, transporting and storing RNA positive controls can be difficult if you don’t have a robust cold-chain established.
This is why Twist partnered with Imagene: to enable broader access to synthetic SARS-CoV-2 RNA and facilitate easier assay development, verification, and validation. Without a need for cold storage, a larger number of encapsulated RNA controls can be stockpiled for a time when rapid access is needed.
“The global COVID-19 pandemic remains a threat in many parts of the world, particularly those countries that have not had access to sufficient quantities of vaccine. In these, and in fact, in all regions of the world, the SARS-CoV-2 virus is fully established and is still a threat to large populations,” said Emily M. Leproust, Ph.D., CEO and co-founder of Twist. “Our synthetic RNA controls help identify, test for, and track SARS-CoV-2 variants. With these new encapsulated versions, we now have the ability to ship and store anywhere in the world, serving every community with a more cost-effective, longer-term solution to help monitor and control this ongoing crisis.”
The longer shelf life, ease of transport and storage, and utility of Twist’s Encapsulated RNA Controls can help laboratories around the world save precious time without sacrificing assay quality.
Learn more about Twist’s Encapsulated RNA Controls.
References
- Oivanen, Mikko, et al. “Kinetics and Mechanisms for the Cleavage and Isomerization of the Phosphodiester Bonds of RNA by Brønsted Acids and Bases.”Chemical Reviews, vol. 98, no. 3, 1998 年 5 月, pp. 961–990, 10.1021/cr960425x.
- Fabre, Anne-Lise, et al. “An Efficient Method for Long-Term Room Temperature Storage of RNA.” European Journal of Human Genetics, vol. 22, no. 3, 1 Mar. 2014, pp. 379–385, www.nature.com/articles/ejhg2013145, 10.1038/ejhg.2013.145.
- Seyhan, Attila A., and John M. Burke. “Mg2+-Independent Hairpin Ribozyme Catalysis in Hydrated RNA Films.” RNA, vol. 6, no. 2, Feb. 2000, pp. 189–198, 10.1017/s1355838200991441.
- “RNAshell®.”Imagene, www.imagene.eu/dnashell-rnashell-en/rnashell-en/. Accessed 11 Apr. 2022.
- Mathay, Conny, et al. “Short-Term Stability Study of RNA at Room Temperature.” Biopreservation and Biobanking, vol. 10, no. 6, Dec. 2012, pp. 532–542, 10.1089/bio.2012.0030.
- Health, Center for Devices and Radiological. “COVID-19 Testing Supplies: FAQs on Testing for SARS-CoV-2.”FDA, 13 Nov. 2021, www.fda.gov/medical-devices/coronavirus-covid-19-and-medical-devices/covid-19-testing-supplies-faqs-testing-sars-cov-2.
Qu’en pensez-vous ?
J’aime
bien
Je n’aime pas
J’aime beaucoup
Je suis surpris(e)
C’est intéressant