12 de diciembre de 2017
Lectura de 7 min
Targeting RNA with CRISPR
A new version of CRISPR targets RNA—rather than DNA—expanding possibilities for new applications.
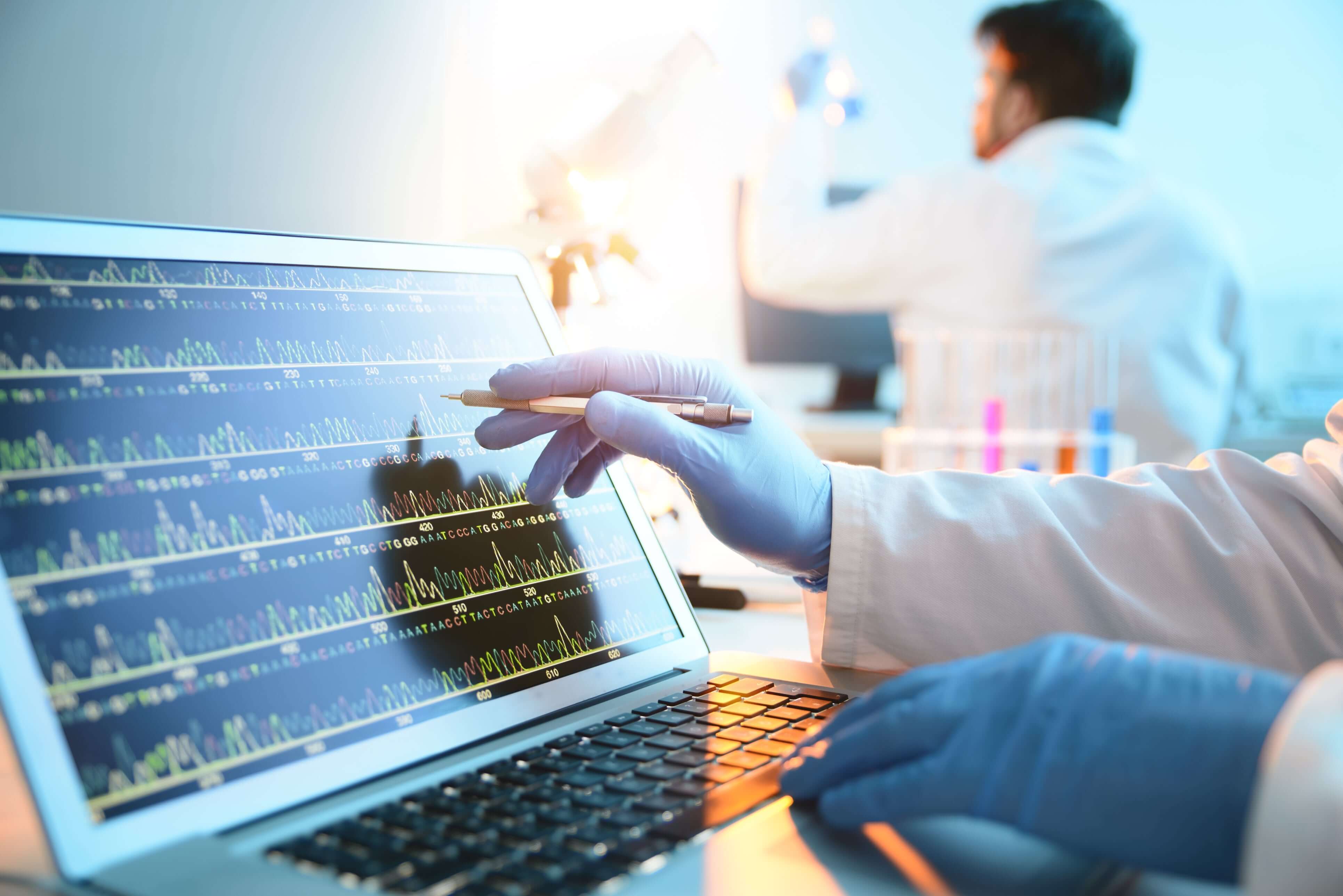
When you hear the word “CRISPR”, you may think of a new technology that allows scientists to quickly and easily edit genomes. However, CRISPR doesn’t stop there—a new version of CRISPR was recently identified that targets RNA—rather than DNA—expanding possibilities for new applications in medicine and biotechnology.
In nature, CRISPR systems in bacteria use RNA derived from repetitive regions of the genome to guide CRISPR-associated proteins to their targets to mediate DNA cleavage. The repetitive regions encoding these RNAs give CRISPR its name—they are called Clustered Regularly Interspaced Short Palindromic Repeats. Each CRISPR-associated enzyme has different properties with respect to both what characteristics of RNAs they prefer for targeting, and how they bind and cut DNA when targeted.
While CRISPR originally evolved as a bacterial defense mechanism to protect against viruses, it can also be harnessed to carry out specific activities in the laboratory. As scientists learn how CRISPR systems function, they are discovering how the systems can be reprogrammed to perform desired activities. For example, once scientists uncover the characteristics of RNA molecules that are responsible for directing cleavage of a specific DNA site, they can create synthetic guide RNAs to target any site of interest, even if such RNAs don’t naturally exist. CRISPR technology allows scientists to mutate a gene in a controlled manner to study its function or repair a faulty gene that causes a disease.
CRISPR technology for targeting and editing genes and genomes is progressing rapidly. Just a few years after discovery, CRISPR experiments are being performed routinely in many different fields. By specifically targeting and cutting DNA, researchers can now use CRISPR to introduce genomic changes in cell lines or animal models in a matter of weeks. Importantly, all of these changes to the genome are permanent—they cannot be reverted to the original genome sequence without another round of editing.
A new discovery from the laboratories of Dr. Feng Zhang and Dr. Eugene V. Koonin may make it possible to create temporary changes to gene expression without altering the genome. Zhang is credited as one of the inventors of CRISPR technology, first showing that mammalian genomes could be edited using CRISPR. Koonin is an evolutionary biologist, skilled in predicting functions of uncharacterized gene products based on their sequence. Their research has identified a new enzyme for the CRISPR toolbox, called C2c2.
Similar to the enzymes in other CRISPR systems, C2c2 is a CRISPR-associated protein that is guided by RNA, but C2c2 is the first to specifically target RNA. As RNA is an intermediate molecule between DNA and protein in gene expression, levels of gene expression can be regulated by targeting RNA using this new CRISPR system. This can be done without permanently editing the genome, allowing researchers to more systematically investigate gene function.
By cutting RNA with C2c2, gene levels can be temporarily decreased. As C2c2 targets RNA rather than DNA, the changes are transient. When C2c2 is no longer present, RNA levels return to normal, and any effect created by the decrease in RNA levels would be reversed. C2c2 doesn’t touch the genome, so any potentially damaging effects disappear after a short time and the changes are not passed on to future generations.
Many diseases are caused by RNA from a certain gene being expressed at high levels. An RNA-based CRISPR system provides the potential to treat such diseases without the long-term risks associated with permanently editing a human genome.
Want to read more? Check out some previous blog posts on a brief history of CRISPR, or some exciting new advances in the CRISPR field.
Characterization of C2c2 Activity
To perform this groundbreaking research, the researchers came to Twist Bioscience to generate an oligo pool consisting of ~3.500 different oligonucleotides representing nearly the entire genome of the phage MS2 (an RNA virus that infects bacteria such as E. coli). This pool was used to generate a library of CRISPR guide RNA molecules. Each guide RNA was a match to a small part of the phage genome, allowing the researchers to investigate which sequences worked best to direct C2c2 to target and cleave the viral RNA.
The screen they performed demonstrated that the C2c2 CRISPR system was functional in E. coli. When C2c2 was targeted to the phage genome by a guide RNA, C2c2 bound and cleaved the RNA genome, protecting the bacterial cells from being killed by the virus. Results of the screen also revealed the guide RNA sequences and structures that cleaved the MS2 RNA genome most efficiently, allowing initial characterization of the RNA-mediated targeting of C2c2.
Researchers then investigated whether the C2c2 system could be reprogrammed to target specific RNAs. This was tested by targeting the mRNA encoding red fluorescent protein (RFP). The C2c2 CRISPR system efficiently reduced expression of RFP, demonstrating that C2c2 could regulate gene expression by targeting specific RNA molecules.
Interestingly, once C2c2 was targeted to cleave a specific RNA sequence, the enzyme also cleaved other “collateral RNAs” non-specifically. The researchers suggested that in nature this may slow down infections enough to allow other antiviral mechanisms to stop the virus from further spreading throughout a population.
What Else is New with C2c2?
More recently, another study on C2c2 from Jennifer Doudna’s laboratory further investigated its biochemical properties and found that C2c2 has two separate enzymatic activities, one for processing the guide RNAs and another for cleaving target RNA molecules.
This study demonstrated that C2c2 is far more unique than originally thought because most CRISPR-associated proteins do not perform both the guide RNA processing and DNA/RNA cleavage steps, and those that do use a completely different enzymatic mechanism.
This newer study also characterized in more detail the non-specific collateral RNA degradation activity that was observed following activation of C2c2. Researchers found that combining C2c2 and a guide RNA with even very small amounts of the target RNA sequence activated the non-specific RNA cleavage.
An additional new report from researchers at the Chinese Academy of Sciences determined the three dimensional structure of the C2c2 protein, both alone and bound to RNA. They found that C2c2 has two distinct and independent RNase catalytic sites, providing an explanation for how C2c2 carries out both specific recognition and processing of CRISPR RNAs and the non-specific collateral degradation of target RNAs. The authors point out that knowing the precise details about how C2c2 binds and cleaves RNA will potentially allow it to be engineered in the future to specifically edit RNA.
Future Applications for C2c2
These new developments in the CRISPR field expand the applications of CRISPR-based technologies and set the stage for the next generation of RNA-specific CRISPR tools for scientific research. The C2c2 system has only been tested in bacterial cells and with human sequences outside of the cells so far, so it will need to be validated in mammalian cells and other model organisms before many potential applications of this technology will be possible.
Additionally, the researchers suggest that the non-specific RNA degradation activity of C2c2 could be adapted to target and kill certain disease cells in humans that express a disease-specific RNA, such as infected cells or cancer cells expressing cancer-associated genes called oncogenes.
While the non-specific RNA degradation activity of C2c2 could be beneficial in certain applications, this additional activity would potentially restrict applications that require the specific targeting of a single RNA molecule. Therefore, thorough characterization of this system is required, and through enzyme engineering it may be possible to mediate the specific RNA cleavage by C2c2 without the associated non-specific cleavage.
In addition to binding and cleaving RNA molecules, the C2c2 CRISPR system has many other potential applications. Researchers have generated a modified version of C2c2 that can bind target RNA but not cleave it, which allows specific programmable RNA-protein interactions. This catalytically inactive version of C2c2 could be fused with a fluorescent protein or an affinity purification tag to investigate RNA localization in cells or to identify interacting DNA, RNA, or proteins. The inactive version of C2c2 could also be fused with an RNA-editing or -modifying enzyme to direct modification of specific RNA molecules.
Parallels can be drawn between the recent work adapting bacteria’s CRISPR adaptive immune system for use in biotechnology and medicine and how we have utilized the mammalian adaptive immune system over the last few decades through the design of antibodies to any target we desire. As new parts of these complex CRISPR systems come to light, it may be possible to reprogram them, building tools that accelerate discovery in molecular biology, biotechnology, and medical research.
¿Qué piensa?
Me gusta
No me gusta
Me encanta
Me asombra
Me interesa
Lea lo siguiente
Suscríbase a nuestro blog y conozca las últimas novedades